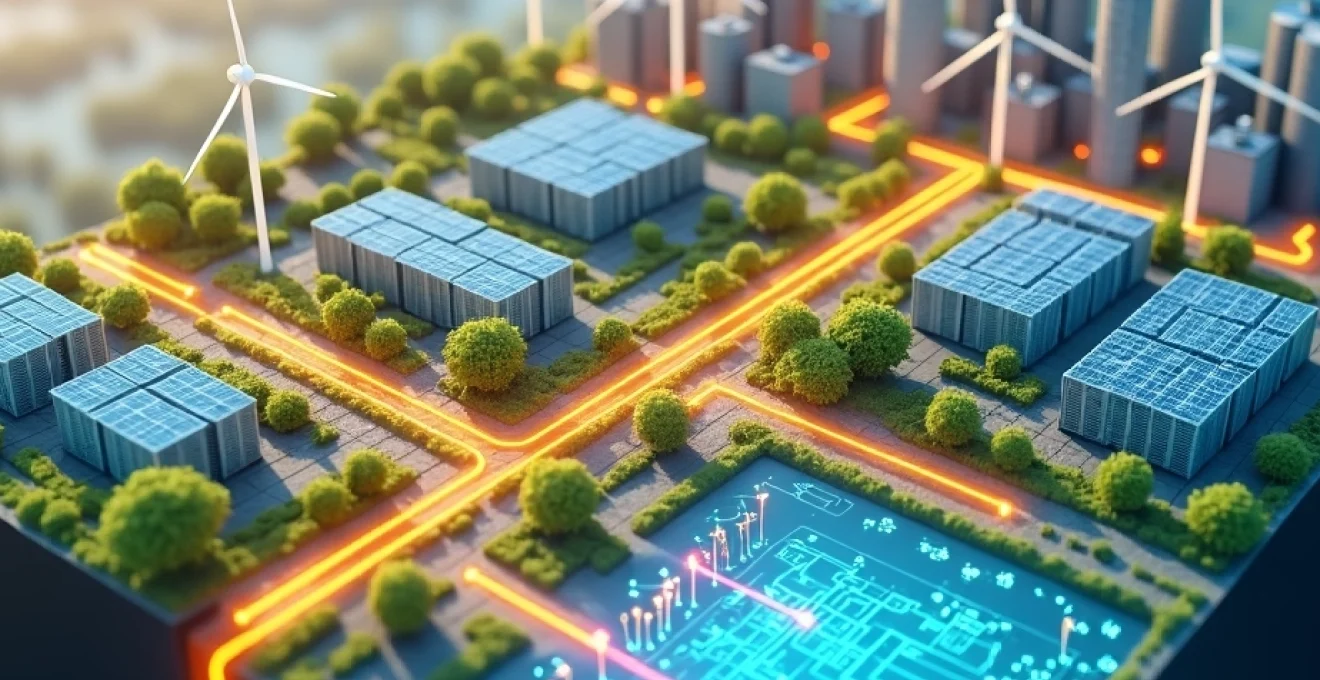
The integration of renewable energy sources into modern power grids represents a critical step towards a sustainable energy future. As the world grapples with climate change and the need to reduce carbon emissions, the transition to renewable energy has become more urgent than ever. This shift, however, presents unique challenges due to the variable nature of many renewable sources like wind and solar power. Overcoming these obstacles requires innovative technologies, smart grid solutions, and supportive policy frameworks to ensure a reliable and efficient energy supply.
Grid integration strategies for variable renewable energy sources
Integrating variable renewable energy (VRE) sources into existing power grids requires careful planning and advanced technological solutions. The intermittent nature of wind and solar power can lead to supply fluctuations, making it challenging to maintain grid stability and meet demand consistently. To address these issues, several strategies have been developed and implemented worldwide.
One key approach is the use of advanced forecasting techniques. By leveraging machine learning algorithms and big data analytics, grid operators can more accurately predict renewable energy generation patterns. This improved forecasting allows for better planning and management of other power sources to complement renewable inputs.
Another crucial strategy is the implementation of flexible generation systems. These include fast-ramping natural gas plants and hydroelectric facilities that can quickly adjust their output to balance the grid when renewable generation fluctuates. This flexibility is essential for maintaining grid stability and reliability in systems with high VRE penetration.
Grid reinforcement and expansion also play a vital role in VRE integration. Upgrading transmission and distribution infrastructure allows for more efficient transport of renewable energy from generation sites to consumption centers. This is particularly important for large-scale wind and solar farms that are often located in remote areas.
Effective grid integration of variable renewable energy sources is not just a technical challenge, but a transformative process that requires a holistic approach encompassing advanced technologies, market design, and regulatory frameworks.
Advanced energy storage technologies for renewable systems
Energy storage is a critical component in the integration of renewable energy sources into modern grids. It addresses the inherent variability of renewables by storing excess energy during peak production periods for use when generation is low. Various advanced storage technologies are being developed and deployed to meet this need.
Lithium-ion battery innovations: Tesla Megapack and beyond
Lithium-ion batteries have emerged as a frontrunner in grid-scale energy storage solutions. The Tesla Megapack, for instance, represents a significant advancement in this technology. These large-scale battery systems can store vast amounts of energy and provide it rapidly when needed, making them ideal for grid stabilization and peak shaving.
Recent innovations in lithium-ion technology have focused on improving energy density, reducing costs, and enhancing safety. Solid-state batteries, which use solid electrolytes instead of liquid ones, promise even greater energy density and improved safety profiles. These advancements are crucial for making large-scale energy storage more economically viable and efficient.
Pumped hydro storage: leveraging topography for energy management
Pumped hydro storage remains one of the most widely used forms of large-scale energy storage globally. This technology uses excess electricity to pump water to a higher elevation reservoir during low demand periods. When energy is needed, the water is released through turbines to generate electricity.
Recent developments in pumped hydro storage include the use of underground reservoirs and seawater systems, expanding the potential locations for these facilities. Additionally, variable-speed pump-turbines have been introduced, allowing for more flexible operation and improved efficiency.
Compressed air energy storage (CAES) in salt caverns
CAES technology stores energy by compressing air in underground caverns during periods of low electricity demand. When energy is needed, the compressed air is released, heated, and expanded through a turbine to generate electricity. Salt caverns are particularly suitable for CAES due to their structural integrity and impermeability.
Advancements in CAES technology include the development of adiabatic systems that capture and store the heat generated during compression, significantly improving overall efficiency. This makes CAES an increasingly attractive option for long-duration energy storage in regions with suitable geological formations.
Flywheel energy storage: high-speed rotational kinetics
Flywheel energy storage systems store energy in the form of rotational kinetic energy. These systems can rapidly absorb or release energy, making them ideal for short-term grid stabilization and frequency regulation. Recent innovations in flywheel technology have focused on reducing friction and increasing rotational speeds to improve energy density and efficiency.
Advanced materials such as carbon fiber composites are being used to construct flywheels capable of withstanding extremely high rotational speeds. Additionally, the use of magnetic bearings and vacuum enclosures has significantly reduced energy losses due to friction, enhancing the overall performance of flywheel systems.
Smart grid technologies enabling renewable integration
Smart grid technologies play a crucial role in facilitating the integration of renewable energy sources into modern power systems. These advanced technologies enable better monitoring, control, and optimization of the grid, allowing for more efficient management of variable renewable energy inputs.
Phasor measurement units (PMUs) for real-time grid monitoring
Phasor Measurement Units, or PMUs, are sophisticated devices that provide real-time, high-resolution measurements of electrical quantities across the power grid. These devices can measure voltage, current, and frequency at a rate of up to 60 times per second, providing grid operators with unprecedented visibility into grid conditions.
The deployment of PMUs enables wide-area monitoring systems (WAMS) that can detect and respond to grid disturbances in real-time. This capability is particularly valuable for managing the variability introduced by renewable energy sources, allowing for faster and more precise control actions to maintain grid stability.
Advanced distribution management systems (ADMS) for renewable coordination
Advanced Distribution Management Systems represent a significant evolution in grid control technology. These integrated software platforms combine multiple functions, including outage management, distribution management, and demand response, into a single, comprehensive system.
ADMS platforms are particularly effective in managing distributed energy resources, including rooftop solar installations and small-scale wind turbines. They enable utilities to optimize power flows, manage voltage levels, and coordinate the operation of various grid assets in real-time, facilitating higher levels of renewable energy integration at the distribution level.
Blockchain-based peer-to-peer energy trading platforms
Blockchain technology is emerging as a potential game-changer in the energy sector, particularly for enabling peer-to-peer (P2P) energy trading. These platforms allow prosumers (consumers who also produce energy) to trade excess renewable energy directly with other consumers in their local grid.
P2P energy trading platforms can help balance local supply and demand, reduce transmission losses, and provide economic incentives for small-scale renewable energy production. By enabling more efficient use of distributed renewable resources, these systems can contribute to greater overall grid stability and resilience.
Ai-driven demand response programs for load balancing
Artificial Intelligence (AI) is revolutionizing demand response programs, making them more dynamic and responsive to real-time grid conditions. AI algorithms can analyze vast amounts of data from smart meters, weather forecasts, and historical consumption patterns to predict demand and optimize load shifting strategies.
These advanced demand response systems can automatically adjust the energy consumption of participating devices (such as smart thermostats or electric vehicle chargers) based on grid conditions and renewable energy availability. This capability helps to balance supply and demand more effectively, facilitating higher levels of renewable energy integration.
Hybrid renewable energy systems: optimizing complementary sources
Hybrid renewable energy systems combine multiple renewable sources to overcome the limitations of individual technologies and enhance overall system reliability. These systems can include combinations of solar PV, wind turbines, hydroelectric power, and various energy storage technologies.
One common hybrid configuration is the solar-wind hybrid system. This setup takes advantage of the complementary nature of solar and wind resources, with solar panels generating power during the day and wind turbines often producing more energy at night or during cloudy conditions. By combining these sources, the system can provide a more consistent power output throughout the day and year.
Another emerging hybrid concept is the integration of floating solar panels with hydroelectric dams. This configuration maximizes land use efficiency and can reduce water evaporation from reservoirs. The solar panels benefit from the cooling effect of the water, which improves their efficiency, while the existing hydroelectric infrastructure can be used for power transmission and storage.
Hybrid renewable energy systems represent a powerful approach to achieving higher renewable energy penetration while maintaining grid stability and reliability. They offer a synergistic solution that can be tailored to local resource availability and energy demand patterns.
Power electronics and inverter technologies for renewable integration
Advanced power electronics and inverter technologies are essential for effectively integrating renewable energy sources into the grid. These technologies facilitate the conversion and control of electrical power, enabling renewable sources to meet grid requirements and contribute to system stability.
Silicon carbide (SiC) and gallium nitride (GaN) power semiconductors
The development of wide-bandgap semiconductors, particularly Silicon Carbide (SiC) and Gallium Nitride (GaN), has revolutionized power electronics for renewable energy systems. These materials offer several advantages over traditional silicon-based semiconductors, including higher operating temperatures, faster switching speeds, and lower losses.
SiC and GaN devices enable the design of more efficient and compact inverters for solar PV systems and wind turbines. Their superior performance characteristics allow for higher power density and improved thermal management, resulting in more reliable and cost-effective renewable energy systems.
Multi-level inverter topologies for high-voltage direct current (HVDC) systems
Multi-level inverter topologies have emerged as a key technology for high-voltage direct current (HVDC) transmission systems, which are increasingly important for connecting large-scale offshore wind farms to onshore grids. These advanced inverter designs can produce a more sinusoidal output voltage with lower harmonic distortion, reducing the need for filtering and improving overall system efficiency.
Modular multi-level converters (MMC) represent a particularly promising technology for HVDC applications. MMCs offer advantages such as scalability, redundancy, and the ability to operate at very high voltages without the need for series-connected devices. These characteristics make them well-suited for long-distance power transmission from remote renewable energy sources.
Fault Ride-Through capabilities in grid-tied inverters
Fault ride-through (FRT) capability is a critical feature of modern grid-tied inverters for renewable energy systems. This functionality allows inverters to remain connected and continue operating during short-term grid disturbances, such as voltage sags or frequency variations. FRT capabilities help maintain grid stability and prevent cascading failures in systems with high renewable energy penetration.
Advanced control algorithms and fast-acting power electronics enable inverters to respond rapidly to grid disturbances, providing reactive power support and helping to stabilize voltage levels. These capabilities are increasingly mandated by grid codes in many countries, reflecting the growing importance of renewable energy sources in modern power systems.
Policy frameworks and market mechanisms supporting renewable integration
Effective policy frameworks and market mechanisms are crucial for facilitating the integration of renewable energy into modern power systems. These structures provide the economic incentives and regulatory support necessary to drive investment in renewable technologies and supporting infrastructure.
Feed-in tariffs (FiTs) have been widely used to promote renewable energy adoption. These policies guarantee a fixed payment for renewable energy fed into the grid, providing long-term revenue certainty for project developers. While effective in spurring initial growth, many countries are now transitioning to more market-based mechanisms as renewable technologies become more cost-competitive.
Renewable portfolio standards (RPS) or renewable energy targets set mandatory requirements for utilities to source a certain percentage of their electricity from renewable sources. These policies create a steady demand for renewable energy and can be designed to increase gradually over time, providing a clear trajectory for the energy transition.
Carbon pricing mechanisms, such as cap-and-trade systems or carbon taxes, are increasingly being implemented to internalize the external costs of fossil fuel generation. These policies create economic incentives for renewable energy by making carbon-intensive generation more expensive, thereby improving the competitiveness of clean energy sources.
Capacity markets and ancillary services markets are evolving to better accommodate renewable energy and energy storage technologies. These markets provide additional revenue streams for renewable projects that can offer grid services such as frequency regulation or voltage support, enhancing the economic viability of these technologies.
Regulatory reforms are also crucial for enabling new business models and technologies that support renewable integration. For example, policies that allow for aggregation of distributed energy resources or peer-to-peer energy trading can help create more flexible and resilient energy systems capable of accommodating high levels of variable renewable energy.
As the energy landscape continues to evolve, policy frameworks and market mechanisms must adapt to address new challenges and opportunities in renewable energy integration. This ongoing process requires close collaboration between policymakers, regulators, industry stakeholders, and researchers to ensure that the regulatory environment keeps pace with technological advancements and changing market dynamics.