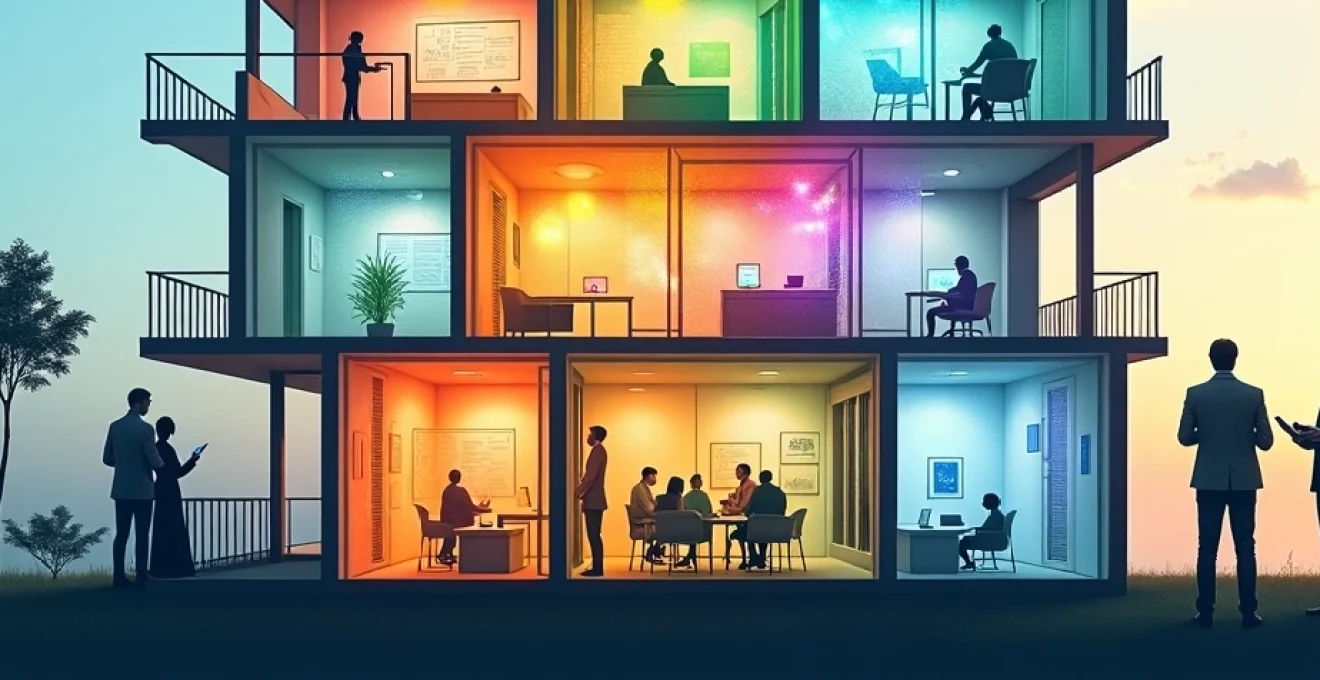
Energy efficiency in buildings has become a critical focus in the era of climate change and resource conservation. As the world grapples with the urgent need to reduce carbon emissions, the built environment offers significant opportunities for improvement. Assessing and optimizing energy efficiency in buildings not only contributes to environmental sustainability but also leads to substantial cost savings for property owners and occupants. This comprehensive exploration delves into the cutting-edge tools and techniques used by professionals to evaluate and enhance building energy performance.
Energy auditing methodologies for building performance analysis
Energy auditing serves as the foundation for understanding a building's energy consumption patterns and identifying opportunities for improvement. These assessments range from basic walk-throughs to detailed analyses using sophisticated equipment and software. Let's examine the various levels of energy audits and the specialized tools employed in this process.
ASHRAE level I, II and III energy audits
The American Society of Heating, Refrigerating and Air-Conditioning Engineers (ASHRAE) has established three levels of energy audits, each providing increasing depth of analysis. A Level I audit, often called a "walk-through audit," involves a brief on-site survey of the facility, a study of utility bills, and a high-level analysis of energy-saving opportunities. This preliminary assessment can help you determine whether a more comprehensive audit is warranted.
Level II audits go a step further, offering a more detailed building survey and energy analysis. These audits typically include an in-depth review of facility operation and maintenance procedures, as well as a thorough analysis of energy bills. Energy conservation measures (ECMs) are identified and evaluated for feasibility and potential savings.
The most comprehensive is the Level III audit, also known as an "investment-grade audit." This detailed analysis provides solid recommendations and financial analysis for major capital investments. It involves rigorous engineering analysis and modeling of energy systems, often using sophisticated software tools like eQUEST or EnergyPlus.
Infrared thermography for heat loss detection
One of the most powerful tools in an energy auditor's arsenal is the infrared camera. This device allows for the visualization of temperature differences across building surfaces, helping to identify areas of heat loss or gain. Infrared thermography can reveal insulation defects, air leakage paths, and thermal bridging issues that might otherwise go unnoticed.
Infrared imaging can detect temperature variations as small as 0.1°C, making it an invaluable tool for pinpointing energy inefficiencies in building envelopes.
When conducting an infrared survey, it's crucial to consider environmental factors such as wind speed, solar radiation, and indoor-outdoor temperature differences to ensure accurate results. The images produced can be analyzed to quantify heat loss and prioritize areas for improvement in the building's thermal envelope.
Blower door testing for air infiltration measurement
Air leakage can account for a significant portion of a building's energy loss. Blower door tests are used to quantify the airtightness of a building envelope and locate specific air leakage paths. This test involves using a powerful fan to depressurize the building, allowing technicians to measure the rate of air infiltration.
The blower door test typically reports results in air changes per hour (ACH) at a standard pressure difference, usually 50 Pascals (ACH50). Many building codes now specify maximum allowable air leakage rates, making blower door testing an essential part of energy efficiency assessments and compliance verification.
Energy modeling software: eQUEST and EnergyPlus
Advanced energy modeling software plays a crucial role in predicting and optimizing building energy performance. Two of the most widely used programs are eQUEST (Quick Energy Simulation Tool) and EnergyPlus, both developed with support from the U.S. Department of Energy.
eQUEST provides a user-friendly interface for creating detailed simulations of building energy use. It allows users to model various building designs and energy efficiency measures, providing comparative analyses of energy consumption and costs. The software is particularly useful for evaluating the impact of different HVAC systems, lighting designs, and building envelope configurations.
EnergyPlus, on the other hand, offers more advanced modeling capabilities and is often used for complex or non-standard building designs. It can simulate heat transfer, thermal comfort, and renewable energy systems with high accuracy. While it has a steeper learning curve than eQUEST, EnergyPlus provides more detailed and customizable outputs, making it a favorite among energy modeling professionals.
Advanced metering and monitoring systems for energy consumption
Accurate measurement and monitoring of energy consumption are essential for identifying inefficiencies and verifying the effectiveness of energy-saving measures. Modern technology has revolutionized the way we collect and analyze energy data, providing unprecedented insights into building performance.
Smart meters and Real-Time energy tracking
Smart meters have transformed the landscape of energy consumption monitoring. Unlike traditional meters that only provide monthly readings, smart meters can record energy use in real-time or at frequent intervals. This granular data allows building managers to identify peak usage periods, unusual consumption patterns, and the impact of specific equipment or activities on overall energy use.
Real-time energy tracking systems often include user-friendly dashboards that display current and historical energy consumption data. These interfaces can be customized to show various metrics, such as kilowatt-hours consumed, cost, and even carbon emissions. By providing immediate feedback on energy use, these systems empower building occupants and managers to make informed decisions about energy consumption.
Building Management Systems (BMS) for energy optimization
Building Management Systems, also known as Building Automation Systems (BAS), are centralized control systems that manage a building's mechanical and electrical equipment. Modern BMS platforms incorporate advanced algorithms and machine learning capabilities to optimize energy use across various building systems.
A sophisticated BMS can integrate data from multiple sources, including occupancy sensors, weather forecasts, and energy prices, to make intelligent decisions about heating, cooling, and lighting. For example, the system might adjust HVAC settings based on predicted occupancy patterns or optimize the use of natural light to reduce artificial lighting needs.
Advanced Building Management Systems can reduce energy consumption by up to 30% through intelligent control and optimization of building systems.
Submetering strategies for granular energy data analysis
While whole-building energy monitoring provides valuable insights, submetering takes energy analysis to the next level. By installing meters on individual circuits, equipment, or areas within a building, facility managers can gain a detailed understanding of where and how energy is being used.
Submetering is particularly useful in multi-tenant buildings or facilities with diverse energy-consuming equipment. It allows for accurate cost allocation and helps identify specific areas or equipment that may be operating inefficiently. Advanced submetering systems can integrate with BMS platforms to provide a comprehensive view of energy use across the entire facility.
Building envelope assessment techniques
The building envelope—comprising walls, roof, windows, and foundations—plays a crucial role in energy efficiency. A well-designed and properly maintained envelope can significantly reduce heating and cooling loads, leading to substantial energy savings. Several techniques are used to assess the performance of building envelopes.
U-Value and R-Value calculations for insulation efficiency
U-value and R-value are key metrics used to quantify the thermal performance of building materials and assemblies. The U-value represents the rate of heat transfer through a material or assembly, with lower values indicating better insulation. Conversely, the R-value measures thermal resistance, with higher values signifying better insulating properties.
Calculating these values involves considering the thermal conductivity of materials, their thickness, and the effects of air films and cavities. For complex assemblies, such as walls with multiple layers, the overall U-value or R-value must be calculated by accounting for all components, including thermal bridging effects.
Air barrier continuity testing methods
An effective air barrier is crucial for preventing uncontrolled air leakage, which can significantly impact energy efficiency and indoor air quality. Testing the continuity of air barriers involves several methods, including visual inspection, smoke testing, and pressurization tests.
One common technique is the use of tracer gas testing, where a non-toxic gas is released inside the building and its concentration is measured over time to determine the rate of air exchange. This method can help identify specific leakage paths and quantify overall air barrier performance.
Window performance evaluation: SHGC and VT ratings
Windows are often the weakest link in a building's thermal envelope. Two key metrics used to evaluate window performance are the Solar Heat Gain Coefficient (SHGC) and Visible Transmittance (VT) ratings.
The SHGC indicates how much solar radiation passes through the window, with lower values meaning less heat gain. This is particularly important in hot climates or for windows with high sun exposure. The VT rating measures the amount of visible light that passes through the window, with higher values indicating more natural light transmission.
Advanced window technologies, such as low-emissivity coatings and gas-filled double or triple panes, can significantly improve both SHGC and VT ratings, leading to better energy performance without sacrificing natural light.
HVAC system efficiency evaluation tools
Heating, Ventilation, and Air Conditioning (HVAC) systems often account for the largest portion of a building's energy consumption. Evaluating and optimizing HVAC efficiency is therefore critical for overall building energy performance. Several metrics and tools are used to assess HVAC system efficiency.
Seasonal Energy Efficiency Ratio (SEER) for air conditioning units
The Seasonal Energy Efficiency Ratio (SEER) is a measure of air conditioning efficiency over a typical cooling season. It is calculated by dividing the total cooling output during normal annual usage by the total electric energy input during the same period. Higher SEER ratings indicate more efficient systems.
Modern high-efficiency air conditioners can achieve SEER ratings of 20 or higher, compared to older units that might have ratings as low as 6 or 8. When evaluating AC units, it's important to consider that actual performance may vary based on climate and usage patterns.
Annual Fuel Utilization Efficiency (AFUE) for heating systems
For heating systems that use combustible fuels, such as natural gas or oil furnaces, the Annual Fuel Utilization Efficiency (AFUE) is the primary measure of efficiency. AFUE represents the percentage of fuel energy that is converted into usable heat over the course of a typical heating season.
Modern high-efficiency furnaces can achieve AFUE ratings of 95% or higher, meaning that 95% of the fuel's energy content is converted into heat for the building. Older or less efficient systems may have AFUE ratings as low as 60-70%, indicating significant energy waste.
Coefficient of Performance (COP) analysis for heat pumps
Heat pumps, which can provide both heating and cooling, are evaluated using the Coefficient of Performance (COP) metric. The COP is the ratio of useful heating or cooling provided to the electrical energy consumed. A higher COP indicates a more efficient system.
For example, a heat pump with a COP of 3 produces three units of heating or cooling energy for every unit of electrical energy consumed. Modern high-efficiency heat pumps can achieve COPs of 4 or higher under optimal conditions. It's important to note that COP can vary significantly based on outdoor temperature and other factors.
Duct leakage testing and sealing protocols
Even the most efficient HVAC equipment can be undermined by leaky ductwork. Duct leakage can result in significant energy waste, reduced comfort, and poor indoor air quality. Testing for duct leakage typically involves pressurizing the duct system and measuring the air flow required to maintain a certain pressure.
The most common method is the "duct blaster" test, which uses a calibrated fan to pressurize the duct system. Leakage rates are typically expressed as a percentage of the total system airflow. Many building codes now specify maximum allowable duct leakage rates, often in the range of 4-6% of system airflow.
Proper duct sealing can improve HVAC system efficiency by 20% or more, leading to significant energy savings and improved indoor comfort.
Lighting systems and daylighting assessment
Lighting can account for a substantial portion of a building's electricity use, making it a prime target for energy efficiency improvements. Assessing lighting systems involves evaluating both artificial lighting and the effective use of natural daylight.
Illuminance measurement techniques and lux meters
Illuminance, measured in lux or foot-candles, is the amount of light falling on a surface. Lux meters are used to measure illuminance levels in various areas of a building. This data helps ensure that lighting levels meet recommended standards for different tasks and spaces while avoiding over-lighting, which wastes energy.
When conducting a lighting assessment, measurements should be taken at various points and times throughout the day to account for changes in natural light. The data collected can be used to create illuminance maps, helping identify areas that are over- or under-lit.
Daylight Factor calculation methods
The Daylight Factor (DF) is a measure of the amount of daylight available inside a room compared to the amount of unobstructed daylight available outside under overcast sky conditions. It is expressed as a percentage and is used to assess the potential for natural lighting in a space.
Calculating the Daylight Factor involves considering factors such as window size and placement, room dimensions, surface reflectances, and external obstructions. While manual calculations are possible, computer simulations using software like Radiance or DIVA for Rhino provide more accurate and comprehensive results, including visualizations of daylight distribution.
LED retrofit analysis tools: DIALux and AGi32
LED lighting retrofits can offer significant energy savings and improved lighting quality. Software tools like DIALux and AGi32 are widely used for planning and analyzing lighting retrofits. These programs allow designers to create detailed 3D models of spaces, specify luminaire types and layouts, and simulate lighting conditions.
These tools can calculate energy consumption, illuminance levels, and other key metrics for both existing and proposed lighting designs. They also provide visualizations that help communicate the expected results of a retrofit to stakeholders. By comparing the performance of different lighting scenarios, designers can optimize for both energy efficiency and lighting quality.
Renewable energy integration and Net-Zero building strategies
As buildings strive for greater energy efficiency and sustainability, the integration of renewable energy systems and strategies for achieving net-zero energy status have become increasingly important. These approaches not only reduce reliance on grid electricity but also contribute to a building's overall energy performance.
Solar PV system sizing and performance modeling
Solar photovoltaic (PV) systems have become a popular choice for on-site renewable energy generation. Sizing a PV system involves analyzing the building's energy consumption, available roof or ground space, local solar resource, and budget constraints. Tools like PVWatts, developed by the National Renewable Energy Laboratory (NREL), provide quick estimates of PV system performance based on location and system characteristics.
More advanced modeling tools, such as SAM (System Advisor Model), allow for detailed performance simulations and financial analysis of solar PV projects. These tools take into account factors like shading, panel orientation, and inverter efficiency to provide accurate predictions of energy production and potential cost savings.
Geothermal heat pump efficiency calculations
Geothermal heat pump systems, also known as ground-source heat pumps, can provide highly efficient heating and cooling by leveraging the relatively constant temperature of the earth. Assessing the feasibility and efficiency of a geothermal system involves considering factors such as soil conditions, available land area, and building heating and cooling loads.
Efficiency calculations for geothermal systems typically use the Coefficient of Performance (COP) metric, similar to air-source heat pumps. However, geothermal systems often achieve higher COPs due to the stable ground temperatures. Specialized software tools, such as GLD (Ground Loop Design), can help designers optimize the sizing and configuration of geothermal loop fields for maximum efficiency.
Energy storage systems: battery capacity and discharge rate analysis
Energy storage systems, particularly battery storage, are becoming increasingly important in building net-zero energy strategies. These systems allow for the storage of excess renewable energy generated during peak production times for use during periods of low production or high demand. Proper sizing and management of energy storage systems are crucial for maximizing their effectiveness and ROI.
When analyzing battery storage systems, key considerations include total capacity (measured in kilowatt-hours), power output (measured in kilowatts), and cycle life. The discharge rate, which is the speed at which a battery can deliver its stored energy, is also critical. This rate is often expressed as a C-rate, where 1C means the battery can be fully discharged in one hour.
Tools like HOMER (Hybrid Optimization Model for Multiple Energy Resources) can be used to model and optimize integrated renewable energy systems, including battery storage. These tools consider factors such as load profiles, renewable resource availability, and electricity tariffs to determine the optimal size and operation strategy for energy storage systems.
Properly sized energy storage systems can increase self-consumption of on-site renewable energy by up to 65%, significantly reducing reliance on grid electricity and improving overall building energy performance.
As buildings strive for net-zero energy status, the integration of renewable energy sources and energy storage becomes increasingly important. Net-zero energy buildings produce as much energy as they consume on an annual basis, achieved through a combination of high-efficiency design and on-site renewable energy generation.
Achieving net-zero status requires a holistic approach, combining passive design strategies, high-performance building envelopes, efficient HVAC and lighting systems, and renewable energy integration. Energy modeling tools play a crucial role in this process, allowing designers to optimize building performance and size renewable energy systems appropriately.